A New Challenge by IHI to Quantitatively Understand Energy Scenarios
Simulating how energy-related technologies will potentially be adopted from an economic perspective
IHI Corporation
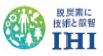
The IHI Group has started a new initiative to utilize economic simulations as it plans to deploy technologies and services related to the environment and energy. Using sustainable aviation fuel (SAF) as an example, we will introduce our initiatives for predicting future energy trends.
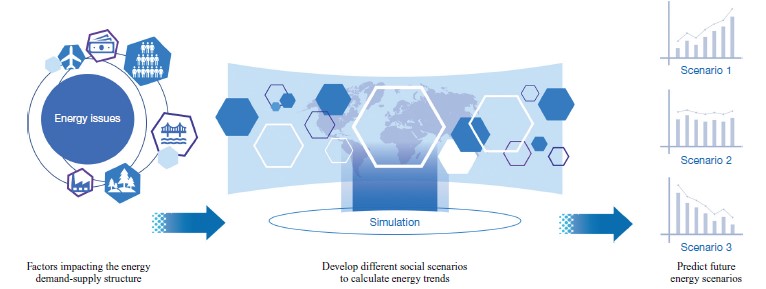
Introduction
Climate change due to global warming has become a social issue around the globe, causing severe impacts on the natural environment such as extreme weather, sea level rise, and changes in ecosystems, and efforts are underway to achieve carbon neutrality. Meanwhile, the energy demand continues to increase, especially in emerging countries. Providing clean energy and resources stably and continuously to all regions is an extremely important social requirement to pursue and maintain a prosperous life for people while protecting the natural environment.
The IHI Group has been providing products and services across the entire energy value chain, including production, conversion, storage, transportation, and utilization. For the future, we need to continue providing environmentally friendly products and services at socially acceptable costs.
As part of efforts to develop such products and services, the IHI Group is working to predict future energy trends through simulations incorporating various factors such as the amount of renewable energy available, global energy demand, the latest technologies related to carbon neutrality, and policy targets for reducing carbon dioxide (CO₂) emissions.
In scenarios where climate measures are implemented strictly versus those where they are implemented moderately, the types of energy to be used and the technologies to be required in future society will be completely different. Thus, by setting different social scenarios and predicting energy trends, we aim to identify the direction of necessary technology development for an uncertain future society.
The complexity of energy issues and energy economics
Quantitative insight into the future transition of the global energy market will allow us to clearly define and develop the products and services and the technologies for creating them.
The energy market changes due to various factors, including global energy demand, advancements in technologies related to carbon neutrality, international rules on CO₂ emission reductions, and the policies of individual countries. Quantitatively capturing future energy trends requires simulations incorporating these factors in the form of parameters.
For example, in developed countries where energy demand does not significantly increase or rather decreases, reducing CO₂ emissions and expanding the use of renewable energy such as solar and wind power contribute to reducing the burden on the global environment and greatly influence energy trends. Meanwhile, in emerging countries where energy demand is expanding, securing power generation facilities and supply chains to stably supply energy significantly affects the trends.
Additionally, Russia’s invasion of Ukraine has substantially changed the energy supply chain especially in Europe and the energy supply-demand structure in Asia. There are regions where solar power generation, wind power generation, and CO₂ storage are not suitable due to weather and geological conditions. The social acceptability of nuclear energy also varies greatly by region. It is required to take the impacts of these events occurring in different regions into consideration and to evaluate global energy trends as a sum of the impacts.
Given that innovative technologies have the potential to significantly advance society toward carbon neutrality, technological progress is also an important factor.
In the IHI Group, we created an overall system incorporating various parameters and are conducting economic simulations to quantitatively evaluate energy scenarios from the perspectives of CO₂ emissions and economic efficiency, predicting future energy scenarios. Based on such findings, we discuss the direction of product and service offerings and technology development.
Methods for energy economy simulation and modeling
Some known methods of modeling energy systems by incorporating social factors and technological advancements include technology selection and general equilibrium models. Analyses are conducted by relating these models to economic and technological premise information.
The technology selection model involves selecting the technology that minimizes the overall system cost under assumed conditions. This model is suitable for quantitatively evaluating which technology to select in scenarios involving fossil fuel-derived, renewable, nuclear energy supply, various energy conversions, and energy use in industrial and transportation sectors.
The general equilibrium model is a model that evaluates the behavior of overall economic activities. This model is useful for capturing the detailed movements of business and economic activities, such as business operations transferring from developed countries to emerging countries due to international regulations, or the shift from energy-intensive manufacturing to energy-saving and low CO₂ emission industries.
In the IHI Group, we mainly use simulations with the technology selection model to broadly explore the possibilities of new products and services needed in future society, and complement this with simulations using the general equilibrium model. Additionally, we consider the technical information relating to design and manufacturing we have been accumulating as a manufacturing company to conduct more accurate analyses.
Simulating global adoption of SAF
Using sustainable aviation fuel (SAF) is considered a promising means of reducing CO₂ emissions in the aviation industry, contributing to a sustainable society. The IHI Group is advancing the study of SAF technology, including its applicability. With the cooperation of the Research Institute of Innovative Technology for the Earth (RITE), we have defined the prerequisites for both social and technical aspects and simulated the scenarios of SAF adoption and the technologies required to promote the adoption by using the technology selection model. In the following section, we will introduce the results of the simulations.
As social prerequisites, we developed two scenarios: one where CO₂ emissions are strictly reduced to limit the global average temperature rise to 1.5°C compared to pre-industrial levels, and another with a more relaxed target of 2.5°C. In addition, we assumed cases where the aviation industry’s goal of switching to carbon-neutral fuels is actively pursued and cases where it is not. In envisioning the world of 2050, we considered scenarios where the ambitious CO₂ emissions reduction target set by the International Civil Aviation Organization (ICAO) to limit temperature rise to 1.5°C is pursued, as well as those where it is not.
As a technical prerequisite for SAF, we assumed the following three types of fuels that vary in raw material and production process. The first is a fuel made from lipid-based biomass such as vegetable oil, produced through hydrogenation (Hydroprocessed Esters and Fatty Acids: HEFA). The second is a fuel produced from biomass raw materials through such processes as alcohol fermentation and dehydration (Alcohol to Jet : ATJ). The third, which is expected to be adopted in the future, is a fuel known as “Power to Liquid (PtL),” which is produced by synthesizing CO₂ and hydrogen made from renewable energy-derived electricity.
We combined these prerequisites with constraints on the amounts of resources used as raw materials, and simulated the technologies and their combinations used to produce aviation fuel in 54 countries and regions worldwide by 2100.
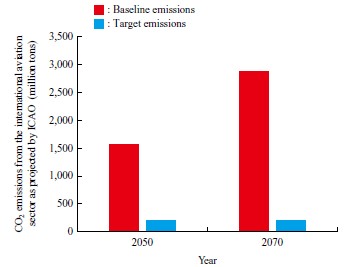
Simulation results on the global adoption of SAF
We calculated SAF production levels worldwide in 2050, under the scenario where the ICAO’s CO₂ emissions reduction target is pursued and the total adoption reaches its maximum. The figure represents the regional differences in annual SAF production volumes using color gradients. The results predicted that approximately 80% of the total annual aviation fuel production of 500 Mtoe (million tons of oil equivalent) would be SAF, with significant production in regions such as North and South America, China, East Africa, and India.
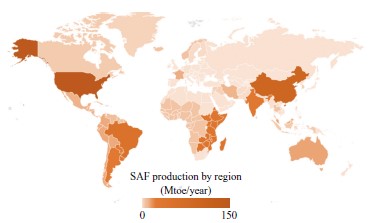
Next, we simulated the three potential patterns in adoption of SAF through the period leading up to 2100. The assumed social scenarios allow us to grasp how the proportion of SAF in the total energy demand and the production volume of different types of SAF will change from a panoramic perspective.
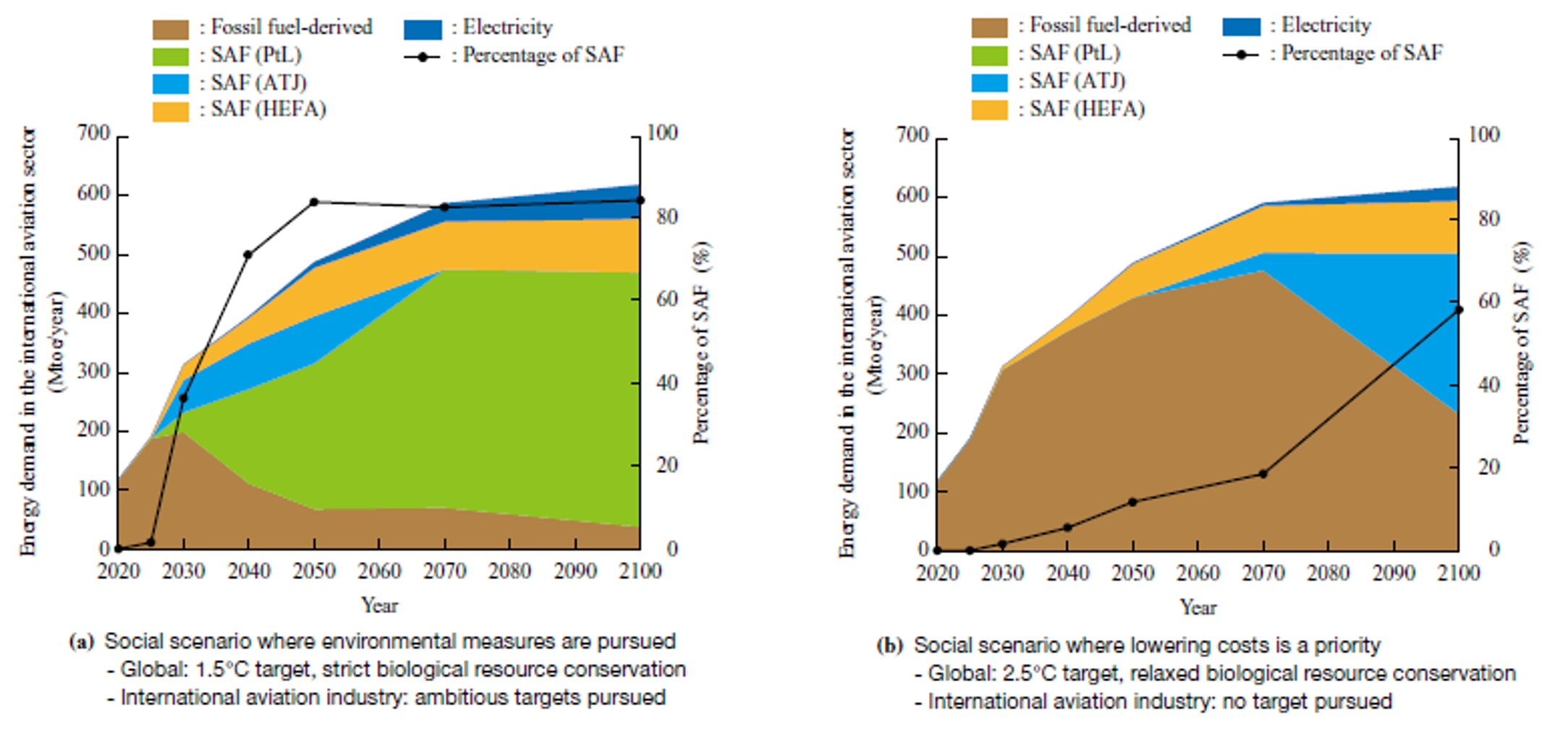
In a social scenario where the goal is to keep the temperature rise to 1.5°C and ambitious environmental measures are pursued globally, including in the aviation industry, ATJ will be relatively common until 2030, as it is a SAF produced from bioethanol at a low cost. The calculations show that the production amount of HEFA, which is inexpensive as it is mainly made from vegetable oil, will reach a certain level, before eventually plateauing due to limited availability of raw materials. Subsequently, the mainstream SAF production technique will shift to PtL, and by 2070, more than half of all SAF will be produced using this technology. In the future, the cost of hydrogen production using renewable energy is expected to decrease significantly, reducing the economic burden and increasing competitiveness. Furthermore, if preserving biological resources is considered a premise of this social scenario, it is suggested that PtL, a technology that uses recovered CO₂ as a raw material, will be more likely to be adopted to mass produce SAF than HEFA or ATJ, which use biomass as a raw material.
In a social scenario aiming for a 2.5°C temperature control target, where the cost-effectiveness of aviation fuel is prioritized over environmental measures, fossil fuel-derived aviation fuel will continue being used. From around 2030 to 2040, production of SAF using HEFA or ATJ will begin, but the adoption of SAF is predicted to remain limited even in 2070.
Consideration of deployment for SAF technology and services
The results obtained from the two SAF adoption scenarios above show that the future SAF adoption rate and SAF manufacturing process will significantly vary depending on society’s stance on tackling climate change.
Additionally, the raw materials and technologies used to produce SAF differ between ATJ, which uses biomass resources, and PtL, which makes SAF from recovered CO₂ and hydrogen. Differences also appear in the regions where SAF is produced, depending on the availability of raw materials used in its production.
We classified the scenarios by technology and region to calculate the SAF production increase in each major region, leading to the findings below.
In a scenario where the temperature control target is set to 1.5°C, PtL, which involves capturing anthropogenic CO₂ emissions and synthesizing fuel from renewable energy, will become more common in regions such as the United States, Europe, and China. Conversely, if proactive measures are not taken under a 2.5°C temperature control target, fossil fuels will continue being used, but an increase in the production through ATJ and HEFA using biomass resources is expected in Africa and South America.
In this way, various predictions can be made through simulations incorporating interrelated factors such as international efforts to combat climate change, the extent of renewable energy adoption, advancements in carbon-neutral technologies, and cost structures. This enables predicting the adoption levels of various types of energy and the technologies used across different social scenarios. The IHI Group will use these predictions to promote SAF-related businesses, technologies and services for society and customers.
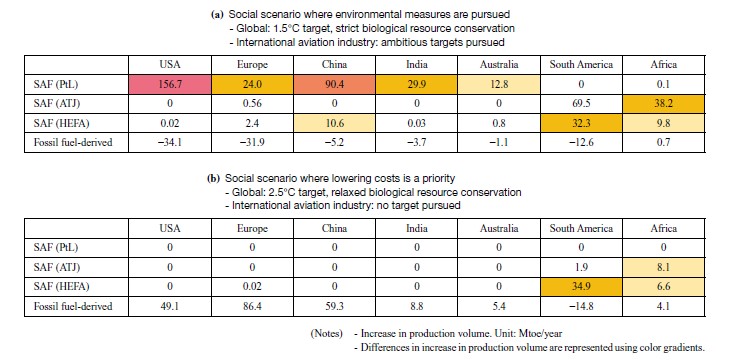
Conclusion
In this article, we introduced an example based on SAF, but we are also simulating the potential adoption of energy-related technologies for fuel ammonia and synthetic methane from an economic perspective in the same way.
The IHI Group will continue to conduct simulations incorporating changes in the social environment to depict multiple scenarios for the future energy market, offering products and services that fit future society.